CNRS Researcher
Electrochemistry - Coordination Chemistry - Spectroelectrochemistry
CNRS Researcher
Etablissement : UMR CNRS UBO 6521
Affectation de recherche : CEMCA (Chimie, électrochimie moléculaires et chimie analytique)
Equipe(s) : CIEL (Inorganic Chemistry and Electrochemistry)

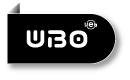
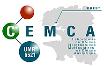
Page personnelle : https://nlepoul.wordpress.com/
Keywords : Electrochemistry, spectroelectrochemistry, small molecules activation (dioxygen, carbon dioxide), surface functionalization, heterogeneous catalysis, supramolecular chemistry.
1. Electrochemistry and spectroelectrochemistry of Cu-oxygen adducts
We have developped room and low temperatures spectroelectrochemical setups (-90°C) which allow in-situ characterization of transient copper-oxygen adducts (superoxo, hydroperoxo, peroxo) and their oxidized or reduced analogues. The latter are believed to be key species involved in fundamental oxidation reactions (H-Atom Transfer, Hydroxylation). The specific setup can also be used for the characterization of many other types of organic and inorganic intermediates.
Associated publications:
Inorg. Chem. 2020, in press. DOI: 10.1021/acs.inorgchem.9b03175.
Chem. Commun. 2019, 55, 12711-12714. DOI: 10.1039/c9cc04422a.
Inorg. Chem. 2018, 57, 12364-12375. DOI: 10.1021/acs.inorgchem.8b02127.
Chem. Commun. 2018, 54, 4931-4934. DOI: 10.1039/C8CC01959B.
Chem. Eur. J. 2018, 24, 5213-5224. DOI: 10.1002/chem.201704613.
Inorg. Chim. Acta 2017, 481, 113-119. DOI: 10.1016/j.ica.2017.09.067.
Chem. Eur. J. 2017, 23, 18314-18319. DOI: 10.1002/chem.201705066.
Chempluschem 2017, 82, 615-624. DOI: 10.1002/cplu.201600636
Chempluschem 2017, 82, 615-624. DOI: 10.1002/cplu.201600636.
Inorg. Chem. 2017, 56, 7707-7719. DOI: 10.1021/acs.inorgchem.7b00338.
Inorg. Chem. 2017, 56, 10971-10983. DOI: 10.1021/acs.inorgchem.7b01225.
Inorg. Chem. 2016, 55, 8263-8266. DOI: 10.1021/acs.inorgchem.6b01504.
Dalton Trans. 2014, 43, 6436-6445. DOI: 10.1039/c3dt53548g.
Dalton Trans. 2013, 42, 2238-2253. DOI: 10.1039/c2dt31456h.
2. Surface functionnalization for sensing and electrocatalysis purposes
Controlled functionalization of electrode surfaces by biomimetic or biological systems is of specific importance for the development of electronic, catalytic, and sensing devices as well as for energy conversion. It also raises the fundamental question of coupling electron transfer to reactivity at a metal active site. An appealing strategy to address this issue consists in the use of self-assembled monolayers (SAMs) thiolato-linked onto a gold electrode and terminated by metal complexes. We have developed a post-funtionalization procedure, based on click chemistry, to graft Cu complexes onto gold electrode as SAMs. The high facility of proceeding and redox behavior of the grafted complex is promising for the immobilization of multi-metallic unsymmetrical systems with fast electron transfer between redox sites.
Associated publications:
J. Am. Chem. Soc. 2016, 138, 12841-12853. DOI: 10.1021/jacs.6b05317.
Langmuir 2014, 30, 4501-4508. DOI: 10.1021/la405005f.
Electrochem. Commun. 2013, 34, 204-207. DOI: 10.1016/j.elecom.2013.06.014.
J. Electroanal. Chem. 2013, 710, 48-58. DOI: 10.1016/j.jelechem.2013.02.014.
Chem. Eur. J. 2012, 18, 594-602. DOI: 10.1002/chem.201102620.
Dalton Trans. 2010, 39, 11516-11518. DOI: 10.1039/c0dt01093f.
3. Redox properties of supramolecular copper complexes
We are investigating the electrochemical properties of different copper complexes bearing a calix[6]arene plaftform mainly for the development of metal-enzyme models. It is now clearly established that secondary coordination sphere effects significantly impact the functionality of metalloproteins (selectivity of the guest molecule, constrained geometries of the metal ion/redox potential, desolvation, stabilization of transient species…). Electrochemical and spectroelectrochemical studies of a series of calixarene complexes have shown that the supramolecular moiety affects the thermodynamics and kinetics of electron transfer coupled to ligand exchange. Recent studies have been focused to the surface functionalization of these complexes for specific aliphatic primay amine detection, or formation of a unique copper-superoxo species.
Associated publications:
Inorg. Chim. Acta 2019, 497, 119081-119090. DOI: 10.1016/j.ica.2019.119081.
Dalton Trans. 2018, 47, 15596-15612. DOI:10.1039/C8DT02952K.
Chem. Sci. 2018, 9, 8282-8290. DOI:10.1039/C8SC03124J.
Inorg. Chem. 2018, 57, 3646-3655. DOI: 10.1021/acs.inorgchem.7b02541.
Dalton Trans. 2017, 46, 15249-15256. DOI: 10.1039/c7dt03375c.
Inorg. Chem. 2017, 56, 10971-10983. DOI: 10.1021/acs.inorgchem.7b01225.
J. Am. Chem. Soc. 2016, 138, 12841-12853. DOI: 10.1021/jacs.6b05317.
Acc. Chem. Res. 2015, 48, 2097-2106. DOI: 10.1021/acs.accounts.5b00152.
Inorg. Chem. 2014, 53, 6224-6234. DOI: 10.1021/ic500740r.
Eur. J. Inorg. Chem. 2013, 2013, 5171-5180. DOI: 10.1002/ejic.201300733.
4. Redox-driven molecular switches
Photochromic organic systems that can undergo substantial variation of their optical properties upon electron stimulus are of high interest for the development of functional materials. In particular, devices based on radical dimerization are well suited because of the effectiveness and speed of the carbon-carbon bond making/breaking. Phenyl-methylenepyrans are organic chromophores which are well suited for such purpose since their oxidation leads to the reversible formation of bispyrylium species. We showed that redox switching for this family of compounds is reversible over several cycles and is accompanied by a significant modification of the UV-Vis spectrum of the chromophore. We have also investigated redox-driven switches based on supramolecular metal complexes. A double translocation (Zn vs Cu) driven by electrochemical input was obtained with a heterobimetallic calix[6]arene dinuclear complex. Also, a “cup-and-ball” redox switch was first demonstrated with a copper-based calix[6]arene system.
Associated publications:
Electrochim. Acta 2019, 305, 304-311. DOI: 10.1016/j.electacta.2019.03.046.
J. Org.Chem. 2017, 82, 12395-12405. DOI: 10.1021/acs.joc.7b02199.
Chemelectrochem 2015, 2, 475-496. DOI: 10.1002/celc.201402399.
Chem. Eur. J. 2013, 19, 10611-10618. DOI: 10.1002/chem.201301141.
J. Am. Chem. Soc. 2010, 132., 4393-4398. DOI: 10.1021/ja910676z